Asymmetry Principle
The Asymmetry Principle was developed by economist Peter Tertzakian, and was first presented in his book The End of Energy Obesity published in 2009. Historically, each energy crisis has brought society to a solutions crossroad: find more or use less. The latter path through conservation efforts has seldom triggered any excitement, so energy shocks have always triggered calls for more supply. With six billion people from the developing world seeking the same living standards as those in the affluent OECD countries the earth’s resources and environment are being put under great strain again. Tertzakian terms this problem the modern world's "energy obesity." In pursuit of a solution, the Asymmetry Principle calls for a shift in focus from energy supply to end use consumption. Within energy economics, Tertzakian highlights how to lower energy consumption without jeopardizing GDP and quality of life.
Introduction
The amount of power and influence we have over energy sources is much greater in the reverse than we realize. Peter Tertzakian calls this influence the Asymmetry Principle of Energy Consumption: “A unit of energy saved at the consumer level cascades into multiple units of energy saved at the source.”[1] Indeed, the size of the savings multiplier, depending on the intended end use for that energy, can be many times over. The proper application of the Asymmetry Principle will provide the leverage society needs to help end “energy obesity”, that is, the profligate and unsustainable consumption of energy in modern society. Not only do we need to better manager and rebalance our energy diet, we need to rebalance our mind-set, too. Tertzakian believes it is possible to resolve our energy issues with a framework that deemphasizes more supply and shifts attention to moderating energy appetite, especially if society is shown the improved lifestyles that are available. The Asymmetry Principle teaches us that real leverage is in the psychology of using less, rather than the technology of using more. We actually command more power when we turn the light switch off instead of on.
Multiples
When you choose not to put energy to work, you save multiples of energy at the point where the primary fuel was sourced. Put another way, the amount of energy saved by not flicking on the wall switch does not translate into an equal amount of energy saved at a coal mine. The leverage up the inefficient supply chain of energy is such that multiples of that energy is saved in terms of the coal not needed to generate your electricity. Consider a simple example. For an incandescent light bulb the multiplier is approximately 50-to-one. A conscious decision to forfeit one quantum of light energy in your home means that 50 times that amount of primary energy is conserved at the source.
Not all examples are as dramatic as lighting, but the multipliers are still very large. For example, one unit of energy saved by not driving a car translates into about six times the amount of energy you no longer need to pump from a wellhead. In other words, the energy equivalent of one barrel of oil saved by not turning wheels means that we can avoid drilling between six and seven barrels of oil in some politically charged, environmentally sensitive, or geographically extreme location.
How does this formula work? The Asymmetry Principle turns one of our deepest problems—inefficient energy use—into our greatest leverage. It is worthwhile to reflect on how shockingly little of the energy we procure from a source like a wellhead or a coal mine actually gets applied in terms of useful work—driving cars, turning on lights, heating homes. The dismaying reality of inefficiencies all along the supply chain means that we throw away most of the energy we secure at the source. But if we reverse that process, we can see how little energy we actually need to conserve in order to save large amounts of primary energy like coal, wind, or oil. In other words, we turn a dismal and stubborn problem into a hopeful and positive solution.
Wasted Resources
Whether it comes from a hydroelectric dam, a big nuclear generator, a coal or gas-fired power plant, or a renewable source, electrical energy follows a similar path through our power distribution infrastructure to meet our needs. You can follow the chain through the main steps from the source to our sockets, to our end use. When we want to turn on the TV or the light switch, a primary source of energy like coal, natural gas, or wind is fed into a device that performs a conversion to high voltage electricity. That electricity is then transmitted along large high-tension wires to a neighborhood substation, where after stepping down the voltage, the electric current travels down smaller wires on the final leg of its journey, either underground or on poles, into your home, typically to a socket in the wall, where it is available for further conversion into the work that you desire. As electricity consumers, we’re almost completely oblivious to that behind-the-scenes process. The power only becomes real when we plug in a blow dryer, vacuum cleaner, light bulb, or television set and convert the electrical energy into useful work. We’re also largely unaware that along the supply chain, there are massive energy losses everywhere. At each stage of conversion or transmission a substantial amount of energy is thrown away, mostly as unused heat. Indeed, the magnitude of the waste we indiscriminately tolerate is such that in most electrical applications, less than 10 percent of the original primary energy is actually for useful work.
Inefficient Power Generation
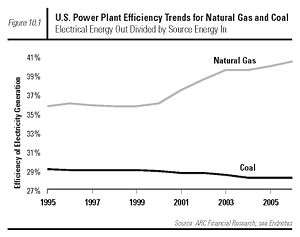
The energy lost from even progressive power generators is substantial. The chain of power generated from a primary fuel like natural gas can be followed. After being piped in from a distant gas field, natural gas is fed into a big stationary turbine, similar to a jet engine bolted to a floor. The gas burns and spins a shaft that is attached to an electrical generator—the modern incarnation of the dynamo. Modern as it is, this primary process is still only able to convert about 40 percent of the energy contained in a cubic foot of natural gas into electricity (United States average). The other 60 percent gets blown out the exhaust as unused heat. Innovations have been made over the past couple of decades and new “combined cycle” generators can now recycle a portion of that wasted heat through another turbine to generate more electrical power in a second stage, bumping up overall efficiency to the 50 to 55 percent range.
Some countries, like Denmark, advance the practice of minimizing waste further by taking even more of the unused heat and putting it to valuable work other than just making electricity. Channeled through a grid of insulated pipes, excess heat is made accessible to nearby buildings for interior climate control. Making good use of both heat and electrical power makes logical sense, driving down waste to 20 percent or less in this first major step of energy conversion.
In reality, achieving average efficiency greater than 50 percent across an entire fleet of natural gas-fired power plants still looks distant in the United States. There are around 5,500 gas-fired power generators across the nation and not all of them are state of the art. Old plants drag the average efficiency down. Nevertheless, progress is being made; since the late 1990s there has been a major buildup of new high-efficiency generators. This modernization has led to a significant improvement in the overall fleet efficiency.
Figure 1 shows that less than 10 years ago only 36 percent of the energy in natural gas was converted to electricity, but that the average has now risen to over 40 percent. This four point efficiency gain is significant: Tertzakian estimates that the positive trend has mitigated the need to bring on close to 1.5 billion cubic feet (42,000,000 m3) a day of new natural gas supply. On average U.S. power plant consumption of 18 billion cubic feet (510,000,000 m3) of gas every day (2008), this curtailment in appetite represents a clear example of how efficiency gains have the potential to help mitigate “energy obesity” on a large scale.
However, this may put a positive spin on natural gas efficiency gains. The sorry truth remains that 60 percent of a non-renewable fuel still goes unused at the power plant, never to be available again. As for coal-fired power plants, there isn’t even a positive trend to cheer. Figure 1 also shows efficiency gains—or rather, lack thereof—for electricity generated from burning coal. It depends on the type of coal being burned, but on average the efficiency of generating electrical power in U.S. coal plants has stalled at 27 percent since 1995, meaning that 73 percent of coal is wasted at the generator—and the journey from the power plant down the wires to the wall socket in your living room has not even begun yet.
As electrons flow down wires, like water through a garden hose, more energy is lost. Those enormous high tension power lines on big metal towers manage to transmit most, but not all, of the electrical energy generated at the power plant. Between 8 and 12 percent vanishes to “line losses,” mostly as heat generated by the resistance of the copper wires. Then, before the electricity is distributed to your home or your business via neighborhood wires, the voltage has to be “stepped down” where yet more losses to resistance ensue. The net result is that only about one third of the electrical energy generated coming out of a power plant whether it’s fueled by coal, natural gas, wood, or wind, makes it to your home.The electricity is now at your wall socket, ready to power a myriad of devices.The stark reality of the numbers will amplify the extent of our inefficiency, but also underscore the magnitude of the potential leverage at our command.
Energy Loses
Consider the simple but enduring incandescent light bulb. The original bamboo-filament light bulbs lit had an efficiency of three percent. That means that only three percent of the electrical energy coming out of the wall socket went to illuminating a room for your eyes to see. Through a series of innovations, most notably the coiled metal filament, the incandescent light bulb had evolved by the mid-1930s into a mature lighting device that hasn’t changed much since. Note that during the winter an incandescent light bulb is actually 100 percent efficient, because the unused heat radiating from the lampshade can serve to provide a small portion of household warmth and comfort. On the other hand, in the summer, the waste heat of all the bulbs forces the air conditioner to work harder to cool down the home, which is doubly wasteful.
Figure 2 catalogs the chained efficiencies in what Tertzakian terms an “asymmetry diagram” and illustrates the cascading energy losses that accrue by the time you flick on the light switch. To simplify, assume that we start with 100 units of energy in the form of natural gas, which is represented by the bar furthest to the left of the diagram.
Big downward steps through the process in Figure 2 represent how many units of energy are left after each stage, and illustrates that the way we put fuels to work is exceptionally wasteful. After the first stage—making electricity through an average U.S. natural gas-fired power plant—only 40 units of the original 100 are left. The next stepdown in Figure 2 reflects power line transmission losses, which eats about five of those 40 units, leaving only 35 for use in the home. While we value light bulbs for their illuminating properties, they perhaps should be more appropriately appreciated as heaters. Ninety percent of the electrical energy a light bulb uses goes to making heat.
The other 10 percent produces light. But our eyes can only see just over half of that light, because our retinas are tuned only to a certain portion of the light spectrum that a bulb emits. That’s why a light bulb is only about six percent efficient at taking electricity from the socket and brightening up a room. So at the end of the process, Figure 2 shows that only two of the original 100 units of natural gas accomplished the desired task: generating visible light.
New coal-fired plants are now achieving close to 40 percent efficiency, but Figure 1 shows that the older plants in the United States bring down the efficiency overall average to 27 percent. Accordingly, the first step-down in the asymmetry diagram is much larger for coal, down to 27 units. In the end, what it means is that for every 100 pounds of coal burned, only 1 pound, 7 ounces is ultimately put to use when lighting a room. Tertzakian's use of the name “asymmetry diagram” becomes clearer now, because you can see that the amount of energy actually put to work at the point of consumption is far less than the original quantity used at the source. In fact, for most energy processes the difference between the potential at the source and the actual use at the end is incredibly lopsided.
Alternative Energy Source of the Future
Our societal issues reside with our energy at the source, for example: the politics of oil, the environmental issues of coal, the scalability and cost of renewables. There is no leverage, that is, no multiplicative benefit to merely adding more supply at the front end of what sustains our society. But that’s what we’ve always done to supply our way out of past energy predicaments and avoid harder choices. Whenever 100 units of fuel have run out, we’ve sought and found another 100 units, or we’ve adopted a different kind of fuel to get those 100 units from an alternative source.
There’s been real resistance to appreciating how the real leverage lies at the consuming end, where the forfeiture of relatively small amounts of energy multiplies into large savings where our biggest problems exist―at the source. This is what the Asymmetry Principle teaches us, and this is our real alternative energy source of the future.
Tertzakian shows how the efficiency stats are dismal. But like the extraordinary offer described here, we can turn the losses between source energy and consumed work into our greatest opportunity. To do so, we need to start thinking about the causality from the opposite direction than we’re accustomed to: from the light bulb toward the coal mine instead of the other way around, from the wheels to the oil well, from the TV set to the wind farm, the furnace to the gas well, and so on. Looking down a cascading asymmetry diagram like Figure 2, a two percent end-to-end efficiency from gas-fired power plant to a light bulb is pathetic, but looking at it in reverse the 50-to-1 leverage is spectacular! That means for every unit of energy not used in lighting, 50 units are forfeited at the gas well.
Even solar power, a limitless green energy source, is incredibly inefficient. If fact, getting energy from sunlight to the light bulb in your house is the most inefficient of all mainstream processes, because a solar panel is only about five percent efficient at the primary conversion stage. Cascading five percent efficiency at your electrical socket through a six percent efficient light bulb, you get a process that only yields 0.3 units of useful work out of 100 units of exposed sunlight. The multiplier here is over 300 times! While sunlight is admittedly plentiful and free, land required for larger-scale solar farms is not. The more solar energy the consumer end user comes to rely on translates into more land we need for solar farms and other space. But if we can find ways to reduce our energy appetites—especially the amount of energy we consume that serves no useful purpose such as unnecessary lighting—the Asymmetry Principle provides a framework of how we can meaningfully cut multiples of that energy at the source, regardless of whether that fuel is green or black.
Looking at all major energy processes in society and ranking the multipliers can serve as a priority list of where the greatest gains can be made. But Tertzakian's purpose here is not to focus on minutiae, but rather to stimulate holistic thinking and methodology. In doing so, we will realize that the magnitude of the inefficiencies in our energy supply chains hands us a unique opportunity to manage our renewable and nonrenewable sources. Boosting system efficiencies (with the caveat of controlling wasteful rebound effects), and reducing end use consumption is far more logical, and has much more potential, than just looking for the next 100 units of energy at the source. Indeed, because there will always be inefficiencies in our energy processes, no technological advances within the energy industry will ever provide us with as much leverage for solving our energy problems as reducing energy consumption.
Presentation
In April, 2010, Peter Tertzakian's ideas on the Asymmetry Principle were presented for dialogue on Canada's national clean energy strategy, in a session hosted by the Canada School for Energy and Environment, the National Round Table on the Environment and the Economy and the Public Policy Forum.
References
- ↑ Peter Tertzakian, The End of Energy Obesity: Breaking Today's Energy Addiction for a Prosperous and Secure Tomorrow (2009). ISBN 0-470-43544-5; ISBN 978-0-470-43544-1.