Auger therapy
Auger therapy (AT) is a form of radiation therapy for the treatment of cancer which relies on a large number of low-energy electrons (emitted by the Auger effect) to damage cancer cells, rather than the high-energy radiation used in traditional radiation therapy.[1][2] Similar to other forms of radiation therapy, Auger therapy relies on radiation-induced damage to cancer cells (particularly DNA damage) to arrest cell division, stop tumor growth and metastasis and kill cancerous cells. It differs from other types of radiation therapy in that electrons emitted via the Auger effect (Auger electrons) are released in large numbers with low kinetic energy. Because of their low energy, these electrons damage cells over a very short range: less than the size of a single cell, on the order of nanometers.[3] This very short-range delivery of energy permits highly targeted therapies, since the radiation-emitting nuclide is required to be inside the cell to cause damage to its nucleus. However, this is a technical challenge; Auger therapeutics must enter their cellular targets to be most effective.[3][4] Auger therapeutics are small molecules, capable of entering cells of interest and binding to specific sub-cellular components, which contain one (or more) heavy atoms capable of emitting Auger electrons by radioactive decay or external excitation.[4]
Auger dose
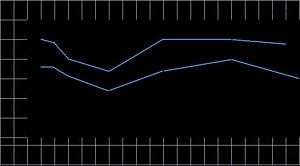
The electron energy in a vacuum may be accurately measured with an electron detector in a Faraday cage, where the bias placed on the cage will accurately define the particle energy reaching the detector. The range of low-energy electrons in tissue or water, particularly electrons at the nanometer scale, cannot be easily measured; it must be inferred, since low-energy electrons scatter at large angles and travel in a zigzag path whose termination distance must be considered statistically and from differential measurements of higher-energy electrons at a much higher range. A 20 eV electron in water, for example, could have a range of 20 nm for 103 Gy or 5 nm for 104.7 Gy. For a group of 9-12 Auger electrons with energies at 12-18 eV in water (including the effect of water ionization at approximately 10 eV), an estimate of 106 Gy is probably sufficiently accurate. The illustration shows the simulated dose calculation in water for an electron using a Monte Carlo random walk[5] which gives up to 0.1 MGy. For a moderately-heavy atom to yield a dozen or more Auger electrons from its inner-shell ionization, the Auger dose becomes 106 Gy per event.
Candidates for molecular modification with in situ dose
With a large, localized dose in situ for molecular modification, the most obvious target molecule is the DNA duplex (where the complementary strands are separated by several nanometers). However, DNA duplex atoms are light elements (with only a few electrons each). Even if they could be induced by a photon beam to deliver Auger electrons, at under 1 keV they would be too soft to penetrate tissue sufficiently for therapy. Mid-range or heavy atoms (from bromine to platinum, for example) which could be induced by sufficiently hard X-ray photons to generate enough electrons to provide low-energy charges in an Auger cascade, will be considered for therapy.
Bromine electrons disrupting herpes-specific gene expression
When a normal cell transforms, replicating uncontrollably, many unusual genes (including viral material such as herpes genes which are not normally expressed) are expressed with viral-specific functions. The molecule proposed to disrupt the herpes gene is BrdC, where Br replaces a methyl (CH3) with nearly the same ionic radius and location (at the 5th position for BrdU, which has an oxygen molecule at the top). Therefore, BrdC could be oxided and used as BrdU. Before oxiding, BrdC was unusable as dC or dU in mammalian cells (except for the herpes gene, which could incorporate the BrdC). The bromine atom is made from arsenic, with the addition of an alpha particle in a particle accelerator to form 77
Br
(with a half-life of 57 hours from its K-electron's capture by a proton from an unstable nucleus. This creates a K hole in Br, leading to its Auger cascade and disrupting the herpes gene without killing the cell.
This experiment was performed during the 1970s at Memorial Sloan Kettering Cancer Center by Lawrance Helson and C. G. Wang, using 10 neuroblastoma cell cultures, Two cultures were successful in terminating the cell replication with 77
Br
in vitro, and the experiments were followed by a group of nude mice with implanted tumors.
The in vivo mouse experiments were complicated when the mouse livers cleaved off the sugar component of BrdC rendering the mammalian and herpes genes to incorporate the 77
Br
-containing base, making no distinction between them. However, the Auger dose with 77BrdC disrupted the herpes-specific gene in several transformed cell cultures.
Lysosome-targeted dose using rose bengal
The stain rose bengal may be ingested with minimal toxicity. Its red molecules contain four iodine atoms each; when they are diffused into cells (particularly transformed cells), they are absorbed by lysosomes attached at the Golgi apparatus. Lysosomes are small sacs of hydrolytic enzymes in the cytoplasm at a pH of 5, which were discovered by Christian de Duve with centrifugation to separate the cellular components.[6]
In normal cellular functions, lysosomes and proteasomes would digest a variety of discarded cellular components or molecules. With rose bengal distributed in the lysosome sac, the Auger dose induced by the inner shell ionization of iodine would disrupt the acidic sacs and alter the pH of the cytoplasm (localized chemotherapy).[4][7]
DNA-targeted dose using cisplatin
The group of metal-based anticancer drugs originated with cisplatin, one of the leading agents in clinical use. Cisplatin acts by binding to DNA, forming one or two intrastrand cross-links of the G-G adduct at 70 % and the A-G adduct at ~20 % of the major grooves of the double helix. The planer cis compound (on the same side) is composed of a square molecule with two chloride ligands on one side and two ammonia ligands on the other side, centered with the heavy platinum (Pt) which could initiate the Auger dose in situ. Entering a cell with a low NaCl concentration, the aqua-chloride ligands would detach from the compound (allowing the missing chloride to link the G-G or A-G bases and bend the DNA helixes 45 degrees, damaging them). Since its discovery in 1978, cisplatin has been modified as carboplatin, nedaplatin and oxaliplatin. Although platinum-based antineoplastics are used in as much as 70 percent of all chemotherapy, they are not particularly effective against certain cancers (such as breast and prostate tumors). The molecular modifications using an in situ megagray Auger dose could alter the DNA helixes in two ways:
- Conventional cancer treatment kills cancer cells rather than altering their transformation process. For cells to replicate, their DNA helixes must be reproduced in each cell cycle using a "zipper" during mitosis. With a megagray in situ Auger dose, the nanoscale damage would probably exceed any single DNA strand (which may be repaired through its complementary strand). With both helix strands damaged, the DNA coding information is lost and the damage is beyond repair. Auger therapy aims to make the "zipper" get stuck (so to speak), aging the cell instead of killing it.
- Radiation and chemotherapy dosages could be reduced. Since the human genome DNA has nine billion base pairs, several breakages anywhere over the molecule could stop the replication process instead of killing the cell. This induced senescence could reduce chemotherapy and radiation doses, reducing side effects, and deliver treatment locally.
The aqua-Cl rationale, detaching the chloride ligands from the cisplatin when they enter a cell and binding them to G-G or A-G adducts in the major grooves of the DNA helixes, could be applied to other metals—such as ruthenium (Ru)-chemically similar to platinum. Ruthenium is used to coat the anode target of a mammography X-ray tube, enabling operation at any voltage (22-28 kVp) depending on the compressed thickness of the breast and delivering a high-contrast image. Although ruthenium is lighter than platinum, it can be induced to provide a megagray Auger dose in situ to the DNA adducts and deliver localized chemotherapy.[8][9]
Gadolinium for localized disruption and deaggregation of beta amyloids
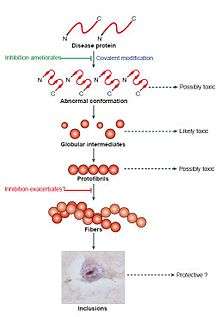
Gadolinium (Gd) has the highest diamagnetic moment in the periodic table, and is used in magnetic resonance imaging (MRI) as a contrast medium; the presence of Gd compounds in MRI from leaky neovascularization has become the gold standard for evaluating metastatic disease.[10] On a nanometer scale, in situ Auger therapy could induce molecular modifications; with a cell dimension of micrometres, an Auger dose could increase the irradiating X-ray dose by a factor of two or three. The enhanced dose may be used as a complement to surgery, "mopping up" local tissue without high-energy, multi-angle gamma beams. Gadolinium is sufficiently heavy for auger therapy, and may be attached to a variety of diagnostic molecules able to bind with the beta amyloids of Alzheimer's disease, for example flutemetamol.[11][12]
Photons reaching the K-absorption edge of gadolinium may be delivered by thulium (Tm) K-emissions using a transmission X-ray tube with a thulium target. The thulium-based X-ray tube would enhance the X-ray scattering cross-section with gadolinium embedded in the target location and deliver 106 Gy in situ for molecular modification (such as the deaggregation of beta amyloids).[13][14]
Monochromatic X-rays to induce inner-shell ionization
X-ray tube with transmission target for line emissions
Monochromatic X-rays may be channeled from synchrotron radiation, obtained from filtered Coolidge X-ray tubes or from the preferred transmission X-ray tubes. To induce inner-shell ionization with resonant scattering from a moderately-heavy atom with dozens of electrons, the X-ray photon energy must be 30 keV or higher to penetrate tissue in therapeutic applications. Although synchrotron radiation is extremely bright and monochromatic without thermal scattering, its brightness falls off at the fourth power of photon energy. At 15-20 kV or higher an X-ray tube with a molybdenum target, for example, could deliver as much X-ray fluence as a typical synchrotron. A Coolidge X-ray tube brightens by 1.7 kVp and synchrotron brightness decreases by 4 kV, implying that it is not useful for Auger therapy.
Although Coolidge X-ray tubes brighten at higher energy, external filtering to harvest useful photons without unnecessarily exposing tissue reduces tube fluence by two or more orders of brightness (not ideal for therapeutic applications). An X-ray tube has been designed with end-window transmission targets.[15]
With a transmission X-ray target, the X-rays are harvested along the direction of the e-beam path, in contrast to a Coolidge tube (where X-rays from a solid target are collected at 90° from the e-beam path). The beam has a sharp cut-off (blocked by the target material) at angles beyond 90°, becoming softer below 90°. The useful X-ray beam from a typical Coolidge tube is 12-14°, although such a narrow cone could be extended along the surface of the solid target to produce a fan beam (commonly used in computed tomography (CT).
Harvesting the X-ray fluence at 90° from the e-beam path is optimal brightness. In relativistic transformation the bremsstrahlung trajectories are forward-leaning, moving along the e-beam; if the X-ray fluence is collected from the transmission target in the forward direction and integrated over the azimuth angles, fluence could be several-hundredfold enhanced compared with the Coolidge tube. The e-beam range in an X-ray target is only a few micrometres; for a 30 μm thick transmission X-ray target, most of the target thickness would absorb low-energy photons and transfer high-energy photons into the fluorescent K- or L-lines characteristic of the target element. Monochromatic line emissions are emitted from the refined X-ray focal point. The illustration demonstrates K-line emissions of molybdenum and silver targets, demonstrating that the transmission target would deliver bright, monochromatic X-rays from the small focal point defined by the e-beam.
Transmission-tube structure
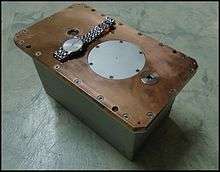
In combining the e-beam target with X-ray filtering, the transmission tube differs from the conventional Coolidge X-ray tube. In a Coolidge tube, the e-beam filament for is at ground, heating a tungsten filament with several amperes of current. A transmission target must be grounded, and the e-beam filament is negatively biased for peak voltage; the several-ampere filament cannot be readily supplied from a high-voltage cable. Therefore, the X-ray transmission tube and its high-voltage power supply are integrated to avoid the use of a high-voltage cable (see illustration).
The transmission X-ray tube could deliver the X-ray beam more efficiently than a tube with a solid e-beam target. The thin target sheet has limited thermal capacity, and must be attached to a thicker, thermally-conductive material transparent to X-rays. Several mm of beryllium is used for end-window material which conducts heat, seals the vacuum and positions the target layer.
The e-beam target layer could also be formed with layers of different materials, each having a different set of line emissions; this would allow the e-beam to be electronically switched to reach the desired layer for the preferred line emission. A tunable line emission could be useful for image manipulation. Although this is a lower-power unit than the proposed X-ray generator for Auger therapy, all are portable.
References
- ↑ Unak, P. (2002) Targeted tumor radiotherapy. Brazilian Archives of Biology and Technology, 45(spe) 97-110.
- ↑ Persson, L. The Auger Electron Effect in Radiation Dosimetry – A Review. Swedish Radiation Protection Institute, S-17116 Stockholm, Sweden.
- 1 2 Kassis, A. (2003) Cancer therapy with Auger electrons: are we almost there? Journal of Nuclear Medicine, 44(9) 1479-1481.
- 1 2 3 Sastry, KSR. Biological effects of Auger emitter iodine-125. A review. Report No. 1 of AAPM Nuclear medicine Task Group No. 6. Med. Phys. 19(6), 1361-1383, 1992.
- ↑ J.Messungnoenab et al, Radiation Research 158, 657-660; 2002
- ↑ de Duve, C. The Lysosome turns 50. Nat. Cell. Biiol. 7(9): 847-849, 2005.
- ↑ Wang, CG and Helson, Lawrence, US Patent 7,981,928 B2; 2011
- ↑ Wang, CG; US Patent 8,278,315; "Radiotherapy Method using X-rays"; 2012.
- ↑ Hannon, Michael J. “Metal-based Anticancer Drugs: From a past anchored in platinum chemistry to a post-genomic future of diverse chemistry and biology”, Pure Appl. Chem. Vol.79, No. 12 pp 2243-2261, 2007.
- ↑ Wilms, G., Marchal, G., Demaerel, PH, Van Hecke, P., Baert, AL. Gadolinium-enhanced MRI of intracranial lesions. A review of indications and results. Clin. Imaging, 15(3): 153-165, 1991.
- ↑ Ikonomovic MD. Et. al. Post-mortem correlates of in vivo PiAB-PET amyloid imaging in a typical case of Alzheimer's disease. Brain 131: 1630-1645, 2008.
- ↑ New Imaging Agent Being Tested for Early Detection of Alzheimer’s. Published online, Diagnostic Imaging. Eli Lilly compound Flutemetamol.
- ↑ Ledford, Heidi. Harmless Prion protein linked to Alzheimer's disease. Published Online 25-Feb-2009; Nature: doi:10.1038/news.2009.121.
- ↑ CA Ross et al., Nature Medicine “Protein Aggregate and Neurodegenerative Diseases” published online July 2004; doi: 10.1038/nm1066
- ↑ Wang, CG, U.S. Patent 7,180,981B2; 2007.
- ↑ Wang, CG and Thomas Vullo, US Patent 7,430,276B2; 2008