Geomagnetic reversal

A geomagnetic reversal is a change in a planet's magnetic field such that the positions of magnetic north and magnetic south are interchanged, while geographic north and geographic south remain the same. The Earth's field has alternated between periods of normal polarity, in which the direction of the field was the same as the present direction, and reverse polarity, in which the field was the opposite. These periods are called chrons.
The time spans of chrons are randomly distributed with most being between 0.1 and 1 million years with an average of 450,000 years. Most reversals are estimated to take between 1,000 and 10,000 years. The latest one, the Brunhes–Matuyama reversal, occurred 780,000 years ago, and may have happened very quickly, within a human lifetime.[1]
A brief complete reversal, known as the Laschamp event, occurred only 41,000 years ago during the last glacial period. That reversal lasted only about 440 years with the actual change of polarity lasting around 250 years. During this change the strength of the magnetic field weakened to 5% of its present strength.[2] Brief disruptions that do not result in reversal are called geomagnetic excursions.
History
In the early 20th century, geologists first noticed that some volcanic rocks were magnetized opposite to the direction of the local Earth's field. The first estimate of the timing of magnetic reversals was made by Motonori Matuyama in the 1920s; he observed that rocks with reversed fields were all of early Pleistocene age or older. At the time, the Earth's polarity was poorly understood, and the possibility of reversal aroused little interest.[3][4]
Three decades later, when Earth's magnetic field was better understood, theories were advanced suggesting that the Earth's field might have reversed in the remote past. Most paleomagnetic research in the late 1950s included an examination of the wandering of the poles and continental drift. Although it was discovered that some rocks would reverse their magnetic field while cooling, it became apparent that most magnetized volcanic rocks preserved traces of the Earth's magnetic field at the time the rocks had cooled. In the absence of reliable methods for obtaining absolute ages for rocks, it was thought that reversals occurred approximately every million years.[3][4]
The next major advance in understanding reversals came when techniques for radiometric dating were developed in the 1950s. Allan Cox and Richard Doell, at the United States Geological Survey, wanted to know whether reversals occurred at regular intervals, and invited the geochronologist Brent Dalrymple to join their group. They produced the first magnetic-polarity time scale in 1959. As they accumulated data, they continued to refine this scale in competition with Don Tarling and Ian McDougall at the Australian National University. A group led by Neil Opdyke at the Lamont-Doherty Geological Observatory showed that the same pattern of reversals was recorded in sediments from deep-sea cores.[4]
During the 1950s and 1960s information about variations in the Earth's magnetic field was gathered largely by means of research vessels. But the complex routes of ocean cruises rendered the association of navigational data with magnetometer readings difficult. Only when data were plotted on a map did it become apparent that remarkably regular and continuous magnetic stripes appeared on the ocean floors.[3][4]
In 1963, Frederick Vine and Drummond Matthews provided a simple explanation by combining the seafloor spreading theory of Harry Hess with the known time scale of reversals: new sea floor is magnetized in the direction of the then-current field. Thus, sea floor spreading from a central ridge will produce pairs of magnetic stripes parallel to the ridge.[5] Canadian L. W. Morley independently proposed a similar explanation in January 1963, but his work was rejected by the scientific journals Nature and Journal of Geophysical Research, and remained unpublished until 1967, when it appeared in the literary magazine Saturday Review.[3] The Morley–Vine–Matthews hypothesis was the first key scientific test of the seafloor spreading theory of continental drift.[4]
Beginning in 1966, Lamont–Doherty Geological Observatory scientists found that the magnetic profiles across the Pacific-Antarctic Ridge were symmetrical and matched the pattern in the north Atlantic's Reykjanes ridges. The same magnetic anomalies were found over most of the world's oceans, which permitted estimates for when most of the oceanic crust had developed.[3][4]
Observing past fields

Past field reversals can be and have been recorded in the "frozen" ferromagnetic (or, more accurately, ferrimagnetic) minerals of consolidated sedimentary deposits or cooled volcanic flows on land.
The past record of geomagnetic reversals was first noticed by observing the magnetic stripe "anomalies" on the ocean floor. Lawrence W. Morley, Frederick John Vine and Drummond Hoyle Matthews made the connection to seafloor spreading in the Morley-Vine-Matthews hypothesis[5][6] which soon led to the development of the theory of plate tectonics. The relatively constant rate at which the sea floor spreads results in substrate "stripes" from which past magnetic field polarity can be inferred from data gathered from towing a magnetometer along the sea floor.
Because no existing unsubducted sea floor (or sea floor thrust onto continental plates) is more than about 180 million years (Ma) old, other methods are necessary for detecting older reversals. Most sedimentary rocks incorporate tiny amounts of iron rich minerals, whose orientation is influenced by the ambient magnetic field at the time at which they formed. These rocks can preserve a record of the field if it is not later erased by chemical, physical or biological change.
Because the magnetic field is global, similar patterns of magnetic variations at different sites may be used to correlate age in different locations. In the past four decades much paleomagnetic data about seafloor ages (up to ~250 Ma) has been collected and is useful in estimating the age of geologic sections. Not an independent dating method, it depends on "absolute" age dating methods like radioisotopic systems to derive numeric ages. It has become especially useful to metamorphic and igneous geologists where index fossils are seldom available.
Geomagnetic polarity time scale
Through analysis of seafloor magnetic anomalies and dating of reversal sequences on land, paleomagnetists have been developing a Geomagnetic Polarity Time Scale (GPTS). The current time scale contains 184 polarity intervals in the last 83 million years.[7][8]
Changing frequency over time
The rate of reversals in the Earth's magnetic field has varied widely over time. 72 million years ago (Ma), the field reversed 5 times in a million years. In a 4-million-year period centered on 54 Ma, there were 10 reversals; at around 42 Ma, 17 reversals took place in the span of 3 million years. In a period of 3 million years centering on 24 Ma, 13 reversals occurred. No fewer than 51 reversals occurred in a 12-million-year period, centering on 15 million years ago. Two reversals occurred during a span of 50,000 years. These eras of frequent reversals have been counterbalanced by a few "superchrons" – long periods when no reversals took place.[9]
Superchrons
A superchron is a polarity interval lasting at least 10 million years. There are two well-established superchrons, the Cretaceous Normal and the Kiaman. A third candidate, the Moyero, is more controversial. The Jurassic Quiet Zone in ocean magnetic anomalies was once thought to represent a superchron, but is now attributed to other causes.
The Cretaceous Normal (also called the Cretaceous Superchron or C34) lasted for almost 40 million years, from about 120 to 83 million years ago, including stages of the Cretaceous period from the Aptian through the Santonian. The frequency of magnetic reversals steadily decreased prior to the period, reaching its low point (no reversals) during the period. Between the Cretaceous Normal and the present, the frequency has generally increased slowly.[10]
The Kiaman Reverse Superchron lasted from approximately the late Carboniferous to the late Permian, or for more than 50 million years, from around 312 to 262 million years ago.[10] The magnetic field had reversed polarity. The name "Kiaman" derives from the Australian village of Kiama, where some of the first geological evidence of the superchron was found in 1925.[11]
The Ordovician is suspected to host another superchron, called the Moyero Reverse Superchron, lasting more than 20 million years (485 to 463 million years ago). But until now this possible superchron has only been found in the Moyero river section north of the polar circle in Siberia.[12] Moreover, the best data from elsewhere in the world do not show evidence for this superchron.[13]
Certain regions of ocean floor, older than 160 Ma, have low-amplitude magnetic anomalies that are hard to interpret. They are found off the east coast of North America, the northwest coast of Africa, and the western Pacific. They were once thought to represent a superchron called the Jurassic Quiet Zone, but magnetic anomalies are found on land during this period. The geomagnetic field is known to have low intensity between about 130 Ma and 170 Ma, and these sections of ocean floor are especially deep, so the signal is attenuated between the floor and the surface.[13]
Statistical properties of reversals
Several studies have analyzed the statistical properties of reversals in the hope of learning something about their underlying mechanism. The discriminating power of statistical tests is limited by the small number of polarity intervals. Nevertheless, some general features are well established. In particular, the pattern of reversals is random. There is no correlation between the lengths of polarity intervals.[14] There is no preference for either normal or reversed polarity, and no statistical difference between the distributions of these polarities. This lack of bias is also a robust prediction of dynamo theory.[10] Finally, as mentioned above, the rate of reversals changes over time.
The randomness of the reversals is inconsistent with periodicity, but several authors have claimed to find periodicity.[15] However, these results are probably artifacts of an analysis using sliding windows to determine reversal rates.[16]
Most statistical models of reversals have analyzed them in terms of a Poisson process or other kinds of renewal process. A Poisson process would have, on average, a constant reversal rate, so it is common to use a non-stationary Poisson process. However, compared to a Poisson process, there is a reduced probability of reversal for tens of thousands of years after a reversal. This could be due to an inhibition in the underlying mechanism, or it could just mean that some shorter polarity intervals have been missed.[10] A random reversal pattern with inhibition can be represented by a gamma process. In 2006, a team of physicists at the University of Calabria found that the reversals also conform to a Lévy distribution, which describes stochastic processes with long-ranging correlations between events in time.[17][18] The data are also consistent with a deterministic, but chaotic, process.[19]
Character of transitions
Duration
Most estimates for the duration of a polarity transition are between 1,000 and 10,000 years;[10] but, some estimates are as quick as a human lifetime.[1] Studies of 15-million-year-old lava flows on Steens Mountain, Oregon, indicate that the Earth's magnetic field is capable of shifting at a rate of up to 6 degrees per day.[20] This was initially met with skepticism from paleomagnetists. Even if changes occur that quickly in the core, the mantle, which is a semiconductor, is thought to remove variations with periods less than a few months. A variety of possible rock magnetic mechanisms were proposed that would lead to a false signal.[21] However, paleomagnetic studies of other sections from the same region (the Oregon Plateau flood basalts) give consistent results.[22][23] It appears that the reversed-to-normal polarity transition that marks the end of Chron C5Cr (16.7 million years ago) contains a series of reversals and excursions.[24] In addition, geologists Scott Bogue of Occidental College and Jonathan Glen of the US Geological Survey, sampling lava flows in Battle Mountain, Nevada, found evidence for a brief, several-year-long interval during a reversal when the field direction changed by over 50 degrees. The reversal was dated to approximately 15 million years ago.[25][26]
Magnetic field
The magnetic field will not vanish completely, but many poles might form chaotically in different places during reversal, until it stabilizes again.[27][28]
Causes
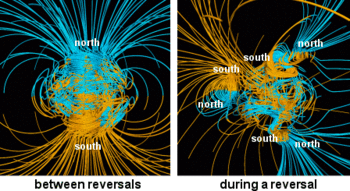
The magnetic field of the Earth, and of other planets that have magnetic fields, is generated by dynamo action in which convection of molten iron in the planetary core generates electric currents which in turn give rise to magnetic fields.[10] In simulations of planetary dynamos, reversals often emerge spontaneously from the underlying dynamics. For example, Gary Glatzmaier and collaborator Paul Roberts of UCLA ran a numerical model of the coupling between electromagnetism and fluid dynamics in the Earth's interior. Their simulation reproduced key features of the magnetic field over more than 40,000 years of simulated time and the computer-generated field reversed itself.[29][30] Global field reversals at irregular intervals have also been observed in the laboratory liquid metal experiment VKS2.[31]
In some simulations, this leads to an instability in which the magnetic field spontaneously flips over into the opposite orientation. This scenario is supported by observations of the solar magnetic field, which undergoes spontaneous reversals every 9–12 years. However, with the Sun it is observed that the solar magnetic intensity greatly increases during a reversal, whereas reversals on Earth seem to occur during periods of low field strength.[32]
Hypothesized triggers
Some scientists, such as Richard A. Muller, think that geomagnetic reversals are not spontaneous processes but rather are triggered by external events that directly disrupt the flow in the Earth's core. Proposals include impact events[33][34] or internal events such as the arrival of continental slabs carried down into the mantle by the action of plate tectonics at subduction zones or the initiation of new mantle plumes from the core-mantle boundary.[35] Supporters of this hypothesis hold that any of these events could lead to a large scale disruption of the dynamo, effectively turning off the geomagnetic field. Because the magnetic field is stable in either the present North-South orientation or a reversed orientation, they propose that when the field recovers from such a disruption it spontaneously chooses one state or the other, such that half the recoveries become reversals. However, the proposed mechanism does not appear to work in a quantitative model, and the evidence from stratigraphy for a correlation between reversals and impact events is weak. There is no evidence for a reversal connected with the impact event that caused the Cretaceous–Paleogene extinction event.[36]
Effects on biosphere
Shortly after the first geomagnetic polarity time scales were produced, scientists began exploring the possibility that reversals could be linked to extinctions. Most such proposals rest on the assumption that the Earth's magnetic field would be much weaker during reversals. Possibly the first such hypothesis was that high energy particles trapped in the Van Allen radiation belt could be liberated and bombard the Earth.[37][38] Detailed calculations confirm that, if the Earth's dipole field disappeared entirely (leaving the quadrupole and higher components), most of the atmosphere would become accessible to high energy particles, but would act as a barrier to them, and cosmic ray collisions would produce secondary radiation of beryllium-10 or chlorine-36. An increase of beryllium-10 was noted in a 2012 German study showing a peak of beryllium-10 in Greenland ice cores during a brief complete reversal 41,000 years ago which led to the magnetic field strength dropping to an estimated 5% of normal during the reversal.[2] There is evidence that this occurs both during secular variation[39][40] and during reversals.[41][42]
Another hypothesis by McCormac and Evans assumes that the Earth's field would disappear entirely during reversals.[43] They argue that the atmosphere of Mars may have been eroded away by the solar wind because it had no magnetic field to protect it. They predict that ions would be stripped away from Earth's atmosphere above 100 km. However, the evidence from paleointensity measurements is that the magnetic field does not disappear. Based on paleointensity data for the last 800,000 years,[44] the magnetopause is still estimated to be at about 3 Earth radii during the Brunhes-Matuyama reversal.[37] Even if the magnetic field disappeared, the solar wind may induce a sufficient magnetic field in the Earth's ionosphere to shield the surface from energetic particles.[45]
Hypotheses have also been advanced linking reversals to mass extinctions.[46] Many such arguments were based on an apparent periodicity in the rate of reversals; more careful analyses show that the reversal record is not periodic.[16] It may be, however, that the ends of superchrons have caused vigorous convection leading to widespread volcanism, and that the subsequent airborne ash caused extinctions.[47]
Tests of correlations between extinctions and reversals are difficult for a number of reasons. Larger animals are too scarce in the fossil record for good statistics, so paleontologists have analyzed microfossil extinctions. Even microfossil data can be unreliable if there are hiatuses in the fossil record. It can appear that the extinction occurs at the end of a polarity interval when the rest of that polarity interval was simply eroded away.[21] Statistical analysis shows no evidence for a correlation between reversals and extinctions.[48][37]
See also
Geomagnetism – Wikipedia book
- Magnetic anomaly
- South Atlantic Anomaly
References
- 1 2 Leonardo Sagnotti, Giancarlo Scardia, Biagio Giaccio, Joseph C. Liddicoat, Sebastien Nomade, Paul R. Renne, Courtney J. Sprain; (21 July 2014). "Extremely rapid directional change during Matuyama-Brunhes geomagnetic polarity reversal". Geophys. J. Int. 199 (2): 1110–1124. Bibcode:2014GeoJI.199.1110S. doi:10.1093/gji/ggu287.
- 1 2 "Ice age polarity reversal was global event: Extremely brief reversal of geomagnetic field, climate variability, and super volcano". Sciencedaily.com. Science Daily. 2012-10-16. Retrieved 2013-07-28.
- 1 2 3 4 5 Cox, Allan (1973). Plate tectonics and geomagnetic reversal. San Francisco, California: W. H. Freeman. pp. 138–145, 222–228. ISBN 0-7167-0258-4.
- 1 2 3 4 5 6 Glen, William (1982). The Road to Jaramillo: Critical Years of the Revolution in Earth Science. Stanford University Press. ISBN 0-8047-1119-4.
- 1 2 Vine, Frederick J.; Drummond H. Matthews (1963). "Magnetic Anomalies over Oceanic Ridges". Nature. 199 (4897): 947–949. Bibcode:1963Natur.199..947V. doi:10.1038/199947a0.
- ↑ Morley, Lawrence W.; A. Larochelle (1964). "Paleomagnetism as a means of dating geological events". Geochronology in Canada. Special. Royal Society of Canada. Publication 8: 39–50.
- ↑ Cande, S. C.; Kent, D. V. (1995). "Revised calibration of the geomagnetic polarity timescale for the late Cretaceous and Cenozoic". Journal of Geophysical Research. 100: 6093–6095. Bibcode:1995JGR...100.6093C. doi:10.1029/94JB03098.
- ↑ "Geomagnetic Polarity Timescale". Ocean Bottom Magnetometry Laboratory. Woods Hole Oceanographic Institution. Retrieved March 23, 2011.
- ↑ Banerjee, Subir K. (2001-03-02). "When the Compass Stopped Reversing Its Poles". Science. American Association for the Advancement of Science. 291 (5509): 1714–1715. doi:10.1126/science.291.5509.1714.
- 1 2 3 4 5 6 Merrill, Ronald T.; McElhinny, Michael W.; McFadden, Phillip L. (1998). The magnetic field of the earth: paleomagnetism, the core, and the deep mantle. Academic Press. ISBN 978-0-12-491246-5.
- ↑ Courtillot, Vincent (1999). Evolutionary Catastrophes: the Science of Mass Extinctions. Cambridge: Cambridge University Press. pp. 110–11. ISBN 978-0-521-58392-3. Translated from the French by Joe McClinton.
- ↑ Pavlov, V.; Gallet, Y. "A third superchron during the Early Paleozoic". Episodes. International Union of Geological Sciences. 28 (2): 78–84.
- 1 2 McElhinny, Michael W.; McFadden, Phillip L. (2000). Paleomagnetism: Continents and Oceans. Academic Press. ISBN 0-12-483355-1.
- ↑ Phillips, J. D.; Cox, A. (1976). "Spectral analysis of geomagnetic reversal time scales". Geophysical Journal of the Royal Astronomical Society. 45: 19–33. Bibcode:1976GeoJI..45...19P. doi:10.1111/j.1365-246X.1976.tb00311.x.
- ↑ e.g., Raup, D. M. (1985). "Magnetic reversals and mass extinctions". Nature. 314: 341–343. Bibcode:1985Natur.314..341R. doi:10.1038/314341a0.
- 1 2 Lutz, T. M. (1985). "The magnetic reversal record is not periodic". Nature. 317: 404–407. Bibcode:1985Natur.317..404L. doi:10.1038/317404a0.
- ↑ Dumé, Belle (March 21, 2006). "Geomagnetic flip may not be random after all". physicsworld.com. Retrieved December 27, 2009.
- ↑ Carbone, V.; Sorriso-Valvo, L.; Vecchio, A.; Lepreti, F.; Veltri, P.; Harabaglia, P.; Guerra, I. "Clustering of Polarity Reversals of the Geomagnetic Field". Physical Review Letters. 96 (12): 128501. arXiv:physics/0603086
. Bibcode:2006PhRvL..96l8501C. doi:10.1103/PhysRevLett.96.128501.
- ↑ Gaffin, S. (1989). "Analysis of scaling in the geomagnetic polarity reversal record". Physics of the Earth and Planetary Interiors. 57: 284–289. Bibcode:1989PEPI...57..284G. doi:10.1016/0031-9201(89)90117-9.
- ↑ Coe, R. S.; Prévot, M.; Camps, P. (20 April 1995). "New evidence for extraordinarily rapid change of the geomagnetic field during a reversal". Nature. 374 (6524): 687. Bibcode:1995Natur.374..687C. doi:10.1038/374687a0.
- 1 2 Merrill, Ronald T. (2010). Our magnetic Earth : the science of geomagnetism. Chicago: The University of Chicago Press. ISBN 0-226-52050-1.
- ↑ Prévot, M.; Mankinen, E.; Coe, R.; Grommé, C. (1985). "The Steens Mountain (Oregon) Geomagnetic Polarity Transition 2. Field Intensity Variations and Discussion of Reversal Models". J. Geophys. Res. 90 (B12): 10417–10448. Bibcode:1985JGR....9010417P. doi:10.1029/JB090iB12p10417.
- ↑ Mankinen, Edward A.; Prévot, Michel; Grommé, C. Sherman; Coe, Robert S. (1 January 1985). "The Steens Mountain (Oregon) Geomagnetic Polarity Transition 1. Directional History, Duration of Episodes, and Rock Magnetism". Journal of Geophysical Research. 90 (B12): 10393. Bibcode:1985JGR....9010393M. doi:10.1029/JB090iB12p10393.
- ↑ Jarboe, Nicholas A.; Coe, Robert S.; Glen, Jonathan M.G. (2011). "Evidence from lava flows for complex polarity transitions: the new composite Steens Mountain reversal record". Geophysical Journal International. 186 (2): 580–602. Bibcode:2011GeoJI.186..580J. doi:10.1111/j.1365-246X.2011.05086.x.
- ↑ Edwards, Lin (6 September 2010). "Evidence of Second Fast North-South Pole Flip Found".
- ↑ Bogue, S.W. (10 November 2010). "Very rapid geomagnetic field change recorded by the partial remagnetization of a lava flow". Geophys. Res. Lett. 37: L21308. Bibcode:2010GeoRL..3721308B. doi:10.1029/2010GL044286.
- ↑ "Earth's Inconstant Magnetic Field". Retrieved 25 Oct 2014.
- 1 2 Glatzmaier, Gary. "The Geodynamo".
- 1 2 Glatzmaier, Gary A.; Roberts, Paul H. "A three dimensional self-consistent computer simulation of a geomagnetic field reversal". Nature. 377. pp. 203–209.
- ↑ Glatzmaier, Gary; Roberts, Paul. "When North goes South".
- ↑ Berhanu, M.; Monchaux, R.; Fauve, S.; Mordant, N.; Petrelis, F.; Chiffaudel, A.; Daviaud, F.; Dubrulle, B.; Marie, L.; Ravelet, F.; Bourgoin, M.; Odier, P.; Pinton, J.-F.; Volk, R. "Magnetic field reversals in an experimental turbulent dynamo". EPL. 77. p. 59001.
- ↑ Coe, Robert S.; Hongré, Lionel; Glatzmaier, Gary A. (2000). "An Examination of Simulated Geomagnetic Reversals from a Palaeomagnetic Perspective". Philosophical Transactions of the Royal Society A: Physical, Mathematical and Engineering Sciences. 358: 1141–1170. doi:10.1098/rsta.2000.0578.
- ↑ Muller, Richard A.; Morris, Donald E. (1986). "Geomagnetic reversals from impacts on the Earth". Geophysical Research Letters. 13 (11): 1177–1180. Bibcode:1986GeoRL..13.1177M. doi:10.1029/GL013i011p01177.
- ↑ Muller, Richard A. (2002). "Avalanches at the core-mantle boundary". Geophysical Research Letters. 29 (19): 1935. Bibcode:2002GeoRL..29s..41M. doi:10.1029/2002GL015938.
- ↑ McFadden, P. L.; Merrill, R. T. (1986). "Geodynamo energy source constraints from paleomagnetic data". Physics of the Earth and Planetary Interiors. 43: 22–33. Bibcode:1986PEPI...43...22M. doi:10.1016/0031-9201(86)90118-4.
- ↑ Merrill, R. T.; McFadden, P. L. (20 April 1990). "Paleomagnetism and the Nature of the Geodynamo". Science. 248 (4953): 345–350. Bibcode:1990Sci...248..345M. doi:10.1126/science.248.4953.345.
- 1 2 3 Glassmeier, Karl-Heinz; Vogt, Joachim (29 May 2010). "Magnetic Polarity Transitions and Biospheric Effects". Space Science Reviews. 155 (1-4): 387–410. Bibcode:2010SSRv..155..387G. doi:10.1007/s11214-010-9659-6.
- ↑ Uffen, Robert J. (13 April 1963). "Influence of the Earth's Core on the Origin and Evolution of Life". Nature. 198 (4876): 143–144. Bibcode:1963Natur.198..143U. doi:10.1038/198143b0.
- ↑ McHargue, L.R; Donahue, D; Damon, P.E; Sonett, C.P; Biddulph, D; Burr, G (1 October 2000). "Geomagnetic modulation of the late Pleistocene cosmic-ray flux as determined by 10Be from Blake Outer Ridge marine sediments". Nuclear Instruments and Methods in Physics Research Section B: Beam Interactions with Materials and Atoms. 172 (1-4): 555–561. Bibcode:2000NIMPB.172..555M. doi:10.1016/S0168-583X(00)00092-6.
- ↑ Baumgartner, S. (27 February 1998). "Geomagnetic Modulation of the 36Cl Flux in the GRIP Ice Core, Greenland". Science. 279 (5355): 1330–1332. Bibcode:1998Sci...279.1330B. doi:10.1126/science.279.5355.1330.
- ↑ Raisbeck, G. M.; Yiou, F.; Bourles, D.; Kent, D. V. (23 May 1985). "Evidence for an increase in cosmogenic 10Be during a geomagnetic reversal". Nature. 315 (6017): 315–317. Bibcode:1985Natur.315..315R. doi:10.1038/315315a0.
- ↑ Raisbeck, G. M.; Yiou, F.; Cattani, O.; Jouzel, J. (2 November 2006). "10Be evidence for the Matuyama–Brunhes geomagnetic reversal in the EPICA Dome C ice core". Nature. 444 (7115): 82–84. Bibcode:2006Natur.444...82R. doi:10.1038/nature05266.
- ↑ McCormac, Billy M.; Evans, John E. (20 September 1969). "Consequences of Very Small Planetary Magnetic Moments". Nature. 223 (5212): 1255–1255. Bibcode:1969Natur.223.1255M. doi:10.1038/2231255a0.
- ↑ Guyodo, Yohan; Valet, Jean-Pierre (20 May 1999). "Global changes in intensity of the Earth's magnetic field during the past 800 kyr". Nature. 399 (6733): 249–252. Bibcode:1999Natur.399..249G. doi:10.1038/20420.
- ↑ Birk, G. T.; Lesch, H.; Konz, C. (2004). "Solar wind induced magnetic field around the unmagnetized Earth". Astronomy & Astrophysics. 420 (2): L15–L18. arXiv:astro-ph/0404580
. Bibcode:2004A&A...420L..15B. doi:10.1051/0004-6361:20040154.
- ↑ Raup, David M. (28 March 1985). "Magnetic reversals and mass extinctions". Nature. 314 (6009): 341–343. Bibcode:1985Natur.314..341R. doi:10.1038/314341a0.
- ↑ Courtillot, V.; Olson, P. (2007). "Mantle plumes link magnetic superchrons to phanerozoic mass depletion events". Earth and Planetary Science Letters. 260. pp. 495–504. doi:10.1016/j.epsl.2007.06.003.
- ↑ Plotnick, Roy E. (1 January 1980). "Relationship between biological extinctions and geomagnetic reversals". Geology. 8 (12): 578. Bibcode:1980Geo.....8..578P. doi:10.1130/0091-7613(1980)8<578:RBBEAG>2.0.CO;2.
Further reading
![]() |
The Wikibook Historical Geology has a page on the topic of: Geomagnetic reversals |
- Behrendt, J.C., Finn, C., Morse, L., Blankenship, D.D. "One hundred negative magnetic anomalies over the West Antarctic Ice Sheet (WAIS), in particular Mt. Resnik, a subaerially erupted volcanic peak, indicate eruption through at least one field reversal" University of Colorado, U.S. Geological Survey, University of Texas. (U.S. Geological Survey and The National Academies); USGS OF-2007-1047, Extended Abstract 030. 2007.
- Okada, M., Niitsuma, N., "Detailed paleomagnetic records during the Brunhes-Matuyama geomagnetic reversal, and a direct determination of depth lag for magnetization in marine sediments" Physics of the Earth and Planetary Interiors, Volume 56, Issue 1-2, p. 133-150. 1989.
External links
- How geomagnetic reversals are related to intensity
- "Look down, look up, look out!", The Economist, May 10 2007
- "Ships' logs give clues to Earth's magnetic decline", New Scientist, May 11 2006
- Simple explanation of geomagnetic reversal, physics.org, accessed Nov 2 2012