Defective interfering particle
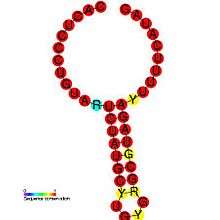
In virology, defective interfering particles (DIPs), also known as defective interfering viruses, are spontaneously generated virus mutants in which a critical portion of the particle's genome has been lost due to defective replication.[2] DIPs are derived from and associated with their parent virus, and particles are classed as DIPs if they are rendered non-infectious due to at least one essential gene of the virus being lost or severely damaged as a result of the defection.[3] A DIP can usually still penetrate host cells, but requires another fully functional virus particle (the 'helper' virus) to co-infect a cell with it, in order to provide the lost factors.[4][5] The existence of DIPs has been known about for decades, and they can occur within nearly every class of both DNA and RNA viruses.[6]
Defection
DIPs are a naturally occurring phenomenon that can also be synthesized for experimental use. They are spontaneously produced by error-prone viral replication, something particularly prevalent in RNA viruses over DNA viruses due to the enzyme used (replicase, or RNA-dependent RNA polymerase.)[3][7] DI genomes typically retain the termini sequences needed for recognition by viral polymerases, and sequences for packaging of their genome into new particles, but little else.[8][9] The size of the genomic deletion event can vary greatly, with one such example in a DIP derived from rabies virus exhibiting a 6.1 kb deletion.[10] In another example, the size of several DI-DNA plant virus genomes varied from one tenth of the size of the original genome to one half.[11]
Interference
The particles are considered interfering when they affect the function of the parent virus through competitive inhibition[3] during coinfection. In other words, defective and non-defective viruses replicate simultaneously, but when defective particles increase, the amount of replicated non-defective virus is decreased. The extent of interference depends on the type and size of defection in the genome; large deletions of genomic data allow rapid replication of the defective genome.[8] During the coinfection of a host cell, a critical ratio will eventually be reached in which more viral factors are being used to produce the non-infectious DIPs than infectious particles.[8]
This interfering nature is becoming more and more important for future research on virus therapies.[12] It is thought that because of their specificity, DIPs will be targeted to sites of infection. In one example, scientists have used DIPs to create "protecting viruses", which attenuated the pathogenicity of an influenza A infection in mice to a point that it was no longer lethal.[13]
Pathogenesis
DIPs have been shown to play a role in pathogenesis of certain viruses. One study demonstrates the relationship between a pathogen and its defective variant, showing how regulation of DI production allowed the virus to attenuate its own infectious replication, decreasing viral load and thus enhance its parasitic efficiency by preventing the host from dying too fast.[14] This also provides the virus with more time to spread and infect new hosts. DIP generation is regulated within viruses: the Coronavirus SL-III cis-acting replication element (shown in the image) is a higher-order genomic structure implicated in the mediation of DIP production in bovine coronavirus, with apparent homologs detected in other coronavirus groups.[1] A more in-depth introduction can be found in Alice Huang and David Baltimore's work from 1970.[15]
Types of defective RNA genomes
- Deletions defections are when a fragment of the template is skipped. Examples of this type of defection can be found in the Resende, et al. 1992 article.[9]
- Snapbacks defections are when replicase transcribes part of one strand then uses this new strand as a template. The result of this can produce a hairpin. Snapback defections are explored in the Schubert & Lazzarini 1981 article.[16]
- Panhandle defections are when the polymerase carries a partially made strand and then switches back to transcribe the 5' end, forming the panhandle shape. Panhandle defections in the influenza virus can be found in the Fodor, et al. 1994 article.[17]
- Compound defections are when both a deletion and snapback defection happens together.
- Mosaic or complex DI genome, in which the various regions may come from the same helper virus genome but in the wrong order; from different helper genome segments, or could include segments of host RNA. Duplications may also occur.[2]
Recent published work
Recent work has been done by virologists to learn more about the interference in infection of host cells and how DI genomes could potentially work as antiviral agents.[2] The Dimmock & Easton, 2014 article explains that pre-clinical work is being done to test their effectiveness against influenza viruses.[18] DI-RNAs have also been found to aid in the infection of fungi via viruses of the family Partitiviridae for the first time, which makes room for more interdisciplinary work.[7]
References
- 1 2 Raman S, Bouma P, Williams GD, Brian DA (2003). "Stem-loop III in the 5' untranslated region is a cis-acting element in bovine coronavirus defective interfering RNA replication". J. Virol. 77 (12): 6720–30. doi:10.1128/jvi.77.12.6720-6730.2003. PMC 156170
. PMID 12767992.
- 1 2 3 Pathak KB, Nagy PD (2009). "Defective Interfering RNAs: Foes of Viruses and Friends of Virologists". Viruses. 1 (3): 895–919. doi:10.3390/v1030895. PMC 3185524
. PMID 21994575.
- ↑ Makino S, Shieh CK, Soe LH, Baker SC, Lai MM (1988). "Primary structure and translation of a defective interfering RNA of murine coronavirus". Virology. 166 (2): 550–60. doi:10.1016/0042-6822(88)90526-0. PMID 2845661.
- ↑ Palmer, S.R. (15 September 2011). Oxford Textbook of Zoonoses: Biology, Clinical Practice, and Public Health Control (2nd ed.). Oxford University Press. pp. 399–400.
- ↑ Stauffer Thompson KA, Rempala GA, Yin J (2009). "Multiple-hit inhibition of infection by defective interfering particles". J. Gen. Virol. 90 (Pt 4): 888–99. doi:10.1099/vir.0.005249-0. PMC 2889439
. PMID 19264636.
- 1 2 Chiba S, Lin YH, Kondo H, Kanematsu S, Suzuki N (2013). "Effects of defective interfering RNA on symptom induction by, and replication of, a novel partitivirus from a phytopathogenic fungus, Rosellinia necatrix". J. Virol. 87 (4): 2330–41. doi:10.1128/JVI.02835-12. PMC 3571465
. PMID 23236074.
- 1 2 3 Dimmock, N. J.; Easton, A. J.; Leppard, K. N. (2007), "13", Introduction to Modern Virology (6th ed.), Oxford, UK: Blackwell Publishing Ltd
- 1 2 Resende Rde O, de Haan P, van de Vossen E, de Avila AC, Goldbach R, Peters D (1992). "Defective interfering L RNA segments of tomato spotted wilt virus retain both virus genome termini and have extensive internal deletions". J. Gen. Virol. 73 ( Pt 10): 2509–16. PMID 1402797.
- ↑ Conzelmann KK, Cox JH, Thiel HJ (1991). "An L (polymerase)-deficient rabies virus defective interfering particle RNA is replicated and transcribed by heterologous helper virus L proteins". Virology. 184 (2): 655–63. doi:10.1016/0042-6822(91)90435-e. PMID 1887588.
- ↑ Patil, Basavaprabhu L.; Dasgupta, Indranil (2006). "Defective Interfering Dnas of Plant Viruses.". Critical Reviews in Plant Sciences. 25 (1): 47–64. doi:10.1080/07352680500391295.
- ↑ Thompson KA, Yin J (2010). "Population dynamics of an RNA virus and its defective interfering particles in passage cultures". Virol. J. 7: 257. doi:10.1186/1743-422X-7-257. PMC 2955718
. PMID 20920247.
- ↑ Easton AJ, Scott PD, Edworthy NL, Meng B, Marriott AC, Dimmock NJ (2011). "A novel broad-spectrum treatment for respiratory virus infections: influenza-based defective interfering virus provides protection against pneumovirus infection in vivo". Vaccine. 29 (15): 2777–84. doi:10.1016/j.vaccine.2011.01.102. PMID 21320545.
- ↑ Lukhovitskaya NI, Thaduri S, Garushyants SK, Torrance L, Savenkov EI (2013). "Deciphering the mechanism of defective interfering RNA (DI RNA) biogenesis reveals that a viral protein and the DI RNA act antagonistically in virus infection". J. Virol. 87 (11): 6091–103. doi:10.1128/JVI.03322-12. PMC 3648117
. PMID 23514891.
- ↑ Huang AS, Baltimore D (1970). "Defective viral particles and viral disease processes". Nature. 226 (5243): 325–7. doi:10.1038/226325a0. PMID 5439728.
- ↑ Schubert M, Lazzarini RA (1981). "Structure and origin of a snapback defective interfering particle RNA of vesicular stomatitis virus". J. Virol. 37 (2): 661–72. PMC 171054
. PMID 6261012.
- ↑ Fodor E, Pritlove DC, Brownlee GG (1994). "The influenza virus panhandle is involved in the initiation of transcription". J. Virol. 68 (6): 4092–6. PMC 236924
. PMID 8189550.
- ↑ Dimmock, N. J.; Easton, A. J. (2014). "Defective interfering influenza virus RNAs: Time to reevaluate their clinical potential as broad-spectrum antivirals?". Journal of Virology. 88 (10): 5217–27. doi:10.1128/JVI.03193-13. PMC 4019098
. PMID 24574404.